Observing the Sun in H-Alpha
H-ALPHA FILTERING SYSTEMS: Unlike continuum “white light” observations of the sun, observing the chromosphere requires a very narrow bandwidth filter centered on the Hydrogen Alpha spectral line (6562.8 Angstroms), which not only reduces the intensity of the sunlight to a safe level, but eliminates much of the photosphere’s contribution to the image. One method of doing this involves using a spectrohelioscope, an image-scanning spectroscope using pairs of moving slits to allow monochomatic viewing of the sun. The instrument has the advantage of rapid tunability not only around H-alpha, but in other spectral lines which show emission, such as the K-line of Calcium. It is rather bulky, and thus is used mainly in a heliostat-fed horizontal solar telescope. For those interested in building one, the basic details are described in the January 1969 issue of Sky and Telescope magazine. The other method of H-alpha viewing involves a special narrow band filter. One design, produced by Lumicon (2111 Research Drive, #5S, Livermore, California 94550), is a 1.5 Angstrom FWHM (Full Width at Half Maximum) Prominence filter, using multi-layer dielectric coatings on a glass surface similar to their nebular filter designs. It is suitable mainly for viewing limb prominences, sunspots, and very bright major flares, since the filter does not have the sharp-edged sub-Angstrom passband needed for revealing much chromospheric disk detail. For better prominence viewing, much more expensive Coronagraph-type viewers are available which use solar occulting disks coupled with a narrow bandpass filter and high-quality optics. For viewing fine chromospheric disk detail, a more complex high quality sub-angstrom bandwidth filter is usually needed. One design, produced by DayStar Filters of California, is a multi-component interference filter using as its core a Fabry-Perot etalon. An etalon is a pair of plane-parallel optical surfaces which are partially reflecting and partially transmitting. With the proper plate spacing, the light undergoes interference, producing a series of very narrow resonant passbands one of which (at the H-alpha line) can be selected via a blocking filter arrangement. These filters have very sharp passband edges, and sub-Angstrom versions allow detailed views of both limb and disk detail. The standard filter stack uses a narrow-band blocking filter, a Fabry-Perot etalon with solid spacer crystal, and a broadband trimming filter. Interference antireflection coatings are placed on the front and back “windows” of the telescope objective for further filtration to protect the filter from excessive solar heating. These filters are quite temperature sensitive, and are often encased in special ovens to keep them within one degree of the required operating temperature (often, they run at over 100 degrees F). Without this control, the passband would wander away from H-alpha fairly quickly. These filters are quite expensive (over $3000), due to the high quality requirements of the quartz elements, and the critical temperature control. They require both electrical power for the ovens, and fairly long f/ratios (f/30 or more) in order to work properly, as the “field angles” of the light hitting the etalon must be very shallow. They are also not rapidly tunable for viewing features in the off-centerline “wings” of H-alpha (unless equipped with a stack-tilt feature). A novel variation on this design is DayStar’s less expensive T-SCANNER 0.7 Angstrom filter. It uses somewhat lower quality quartz, operates from 32 to 104 degrees F (0 to 40 deg. C), and deals with the temperature control problem by using an adjustable tilt in the filter stack to change the optical path length through the filter. Not only does this variable tilt allow the user to compensate for temperature variations, but it also makes possible rapid tuning of the filter for viewing Doppler-shifted features. The tiltable stack also means no filter heater electrical power or warm-up time is needed. This, plus the lower quality quartz elements cuts the cost of the filter nearly in half. It still requires at least an f/30 light cone, but this can be easily achieved with auxiliary lenses, or by stopping down larger apertures. However, the use of a standard barlow to achieve f/30 in an off-axis stopped-down Newtonian Telescope may only allow part of the field of view to be in the passband at any one time. This is the so-called “ring” effect, in which the area of the field which is in the passband is shaped like a thick annular ring. At low ambient temperatures (near the low temperature operating limit of 32F), this ring contracts into a broad disk of H-alpha detail, and at temperatures near the filter’s upper operating limit, the ring expands into a broad low-contrast arc. However, using Tele Vue’s 2x or 2.5x Powermate instead of a Barlow to achieve the f/30 or more will allow most of the full field to be in the passband. The contrast of the image is also somewhat higher when the filter is cool (closer to its lower temperature limit of 32 degrees F.)
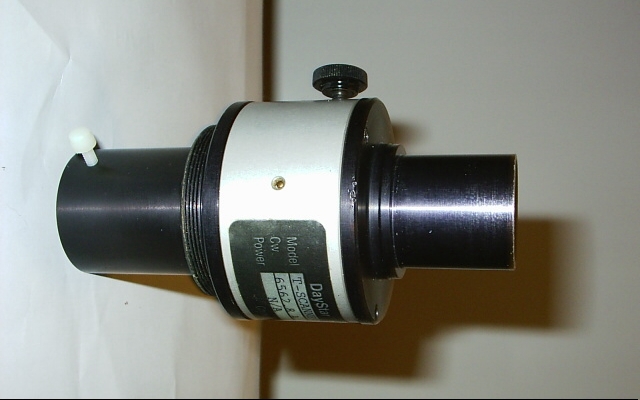
Many telescopes can be adapted to work fairly well with this filter, and the aperture does not have to be very large for visual observations (50 to 150 mm typically). Contrast for visual observation is lower than that of the heated high quality filters, but is acceptable, and is sufficient for TV camera operation. The smaller camcorders can be quite useful, as they can be easily coupled to many eyepieces, and can enhance details which can’t be seen as easily visually. They can also let more than one person at a time view the sun, and can record the view for later educational viewing and study. A good thermally-stable observing site is a must, since daytime seeing problems can virtually wipe out much of the fine disk detail. More recently, sub- angstrom higher quality oven-heated filters from DayStar have also been equipped with tilt to allow them to be more rapidly tuned, although they are still more expensive than the T-Scanner. Another etalon-based design of H-alpha filter which have the etalon placed over the front of the telescope objective are also now being produced by Coronado Instruments (Tuscon, Arizona). This out-front placement avoids the field-angle problems and some of the thermal control problems that filters placed near the telescope focus tend to have. However, in order to get much of an aperture, the etalon must be made considerably larger than that needed for the near-focus etalon-based filters, so the “out-front” Coronado designs tend to be considerably more expensive. A novel introductory H-alpha telescope, the “PST” or “Personal Solar Telescope” has been introduced by Coronado, and despite its 40mm aperture, it delivers fine performance with its built-in H-alpha filter.
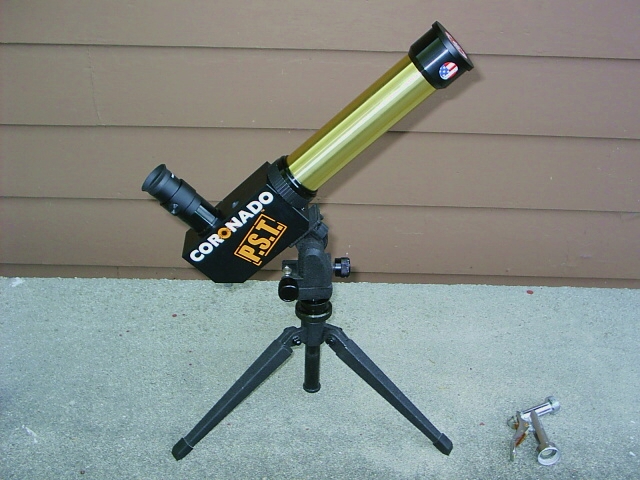
For more information on H-alpha filters, see ASTROPHYSICS OF THE SUN, by Harold Zirin, p. 23-29, or Amateur Telescope Making, Book III, p. 376-428. ****************************************************************************
GLOSSARY OF MAJOR H-ALPHA TERMS (* indicates a term which is generally not an H-alpha feature, but which is mentioned in solar texts).
CHROMOSPHERIC NETWORK: An ever-present patchy network of long thin sinuous chains of tiny low contrast brighter points called Filigree (also found in plages) extending over much of the solar disk in H-Alpha. These points, or “network elements”, often have darker spicules or short fibrils sticking out of, or running past them (part of the fine disk detail known as the Dark Mottles), making the actual network harder to see.
ELLERMAN BOMBS: Tiny fairly bright transient points of light (usually last less than 5 minutes), most often found in Emerging Flux Regions or on the edges of sunspots where the magnetic field is breaking the surface. They are best seen in the wings of H-alpha (nearly 5 Angstroms wide).
EMERGING FLUX REGION (EFR): An area on the sun where a magnetic dipole, or “flux tube” is surfacing on the disk, eventually producing a bipolar sunspot group. In H-alpha, EFRs usually appear as a small oval area of bright plage (typically about 7000 km across) often containing a series of short-lived narrow fibrils (Arch Filament System (AFS)) running roughly from one end of the dipole to the other. Each pole of an EFR is often marked by pores or small developing sunspots. Surges or even small solar flares can sometimes occur in EFRs.
EPHEMERAL REGIONS (ER’s): Small magnetic dipoles with lifetimes of about a day which contain no sunspots. Ephemeral regions can develop anywhere on the sun, but are more common at mid and lower solar latitudes. They appear as small brighter elements in the chromospheric network but are fainter than active region plage. They also can occasionally produce small surges or subflares.
*FACULAE: Patchy white light brightenings in the photosphere (not visible in H-alpha), usually seen mainly towards the limb due to limb darkening. Faculae are most often found near active regions or where one is about to form, and can last well after the sunspots in the active region have decayed (best seen in blue light).
FIBRILS: Small fine filament-like darker features which tend to run along magnetic field lines. Often, they are connected to or part of the structure of larger filaments, curving into or running along the filament’s main axis.
FIELD TRANSITION ARCHES (FTA): Filament-like fibrils which cross the polarity inversion line (a line marking the halfway point between two opposite polarity areas) of a bipolar magnetic region. Unlike AFS fibrils, they show little or no Doppler shifts and tend to be rather thin and not very dark. FTA tend to arch directly between localized areas of opposite magnetic polarity, and often mark magnetically stable regions.
FILAMENTS: Prominences seen against the face of the sun, appearing as long narrow dark streamers or diffuse complex dark areas in H-alpha light. Filaments often mark areas of magnetic shearing (see Prominences).
*GRANULATION: Small scale convective cell structures visible in white light (“rice grains”), best seen in apertures over three inches, and in green light. Each cell consists of a brighter polygonal area of hot rising gas typically about 1100 km across, and a cooler edge or “channel” of descending gas about 230 km wide.
MORETON WAVE: A chromospheric shock wave that is sometimes seen expanding outward from large impulsive solar flares, moving over the surface at about 1000 km/sec. It usually appears as a slowly moving diffuse arc of brightening in the centerline of H-alpha, or as a faint diffuse slightly darker arc in the blue wing.
PROMINENCES: H-Alpha emission features projecting beyond the limb of the sun, consisting of complex clouds or streamers of gas above or in the chromosphere. They generally come in two broad classes: Active (limb flares, surges, sprays, loops), and Quiescent (Quiet Region Filaments, Active Region Filaments).
PLAGE: Patchy H-Alpha brightenings on the solar disk, usually found in or near active regions, which can last for several days. Plage is irregular in shape and variable in brightness, marking areas of nearly vertical emerging or reconnecting magnetic field lines (from French word for “beach” with the “a” being a short one).
PORES: Tiny darker spots under 2500 km in size, often having fairly short lifetimes. Pores occasionally form where several granulation channels meet and can sometimes precede the development of sunspots.
RECONNECTION: A realignment of magnetic fields, where an area of one magnetic polarity breaks eariler links, and connects with the nearest region of opposite polarity. On the sun, this often happens when a new magnetic dipole emerges near another pre-existing one. For example, if the north pole of the new dipole emerges close to the south pole of the old dipole, the lines of force may reconnect these two nearby poles configuring them as a new lower energy dipole and releasing energy, often in the form of plage brightening or a solar flare.
SOLAR FLARE: Extremely bright moderate to large transient emission feature lasting from a few minutes to over four hours. Flares are a rapid and violent release of energy in the chromosphere due to extreme magnetic field stress and can occasionally result in material leaving the sun in the form of a Coronal Mass Ejection (CME).
SPICULES: Small jets of gas under 10,000 km long, usually seen as a mass of tiny brighter spike-like features at the limb or as tiny darker spikes coming out of network elements, but are not usually seen over bright plage.
SPRAY: A transient prominence formed by the explosion of pre-flare elevated material which sends debris flying off in many directions. Usually produced only by the most violent flares, as overlying filaments are blown away.
SUNSPOT: Dark long-lived photospheric feature, typically from 2500 to 50,000 km in size. Moderate to large spots usually consist of a darker central region (umbra) and a lighter halo consisting of many short fine fibrils (penumbra). Sunspots have strong concentrated magnetic fields which tend to inhibit energy transfer from below, making them at the center about 2500 degrees K cooler than the photosphere. In the Umbra, the fields tend to be nearly vertical in orientation while in the penumbra, the magnetic fields become more horizontal.
SURGE: A transient prominence produced by flares or very active regions, appearing as a moderate to large collimated jet of gas rising up from the surface. Surge ejected gas will often fall or draw back onto the sun tending to follow magnetic field lines, while at other times it will rise and disperse, fading from view.
“WINGS” OF H-ALPHA: Wavelengths slightly off of 6562.8 Angstroms (up to +/- 2 Angstroms), used for viewing Doppler-shifted features. The “blue” wing is a shorter wavelength and the “red” is on the longer side.
SOLAR PROMINENCES
Prominences are huge clouds of gas which are often seen on or above the solar limb, and are the easiest H-alpha emission feature to observe, requiring a filter passband that can be one or two Angstroms wide. Prominences observed on the solar disk are the darker features known as Filaments, and need a passband width under one Angstrom in order to be clearly visible. They often represent a sheared magnetic field boundary or neutral line between opposite polarities where gas is trapped around bunched field lines. The forms prominences can take vary widely, but the following general scheme (Zirin) is somewhat useful in categorizing them:
CLASS 1: QUIESCENT FILAMENTS/PROMINENCES (long lived, fairly static) a. QRF (Quiet Region Filament) ie: hedgerow, curtains, floating arches, arcs, fans, ect. b. ASCENDING PROMINENCES (end of the quiescent phase) “Disparition Brusque” (“lifting off”) eruption. c. ARF (Active Region Filament): a filament in or near an active region (netural line filaments).
CLASS 2: ACTIVE FILAMENTS/PROMINENCES (short-lived, moving) a. LIMB FLARES (bright blobs, sometimes expanding into eruptive prominences). b. SURGES: collimated ejected material previously not seen (very sudden jet of gas). c. SPRAYS: uncollimated ejecta previously visible as pre-flare elevated features (violent explosion). d. FLARE LOOPS and CORONAL RAIN (often post-flare ejecta or other gas).
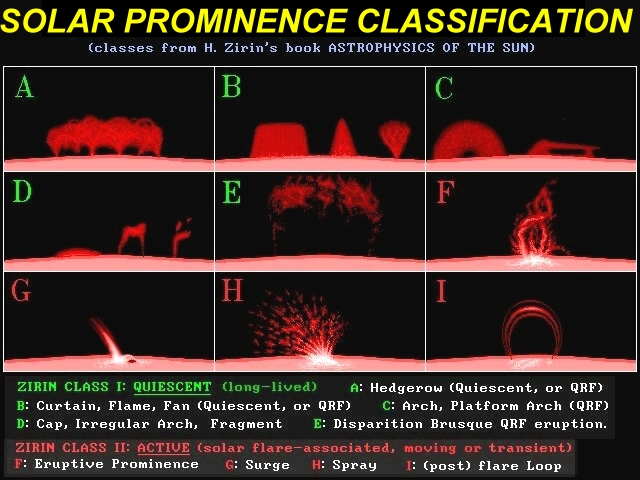
QUIESCENT PROMINENCES/FILAMENTS take a wide variety of forms, and tend to be much longer than they are wide. Quiet Region Filaments (QRFs) are some of the largest and longest lasting quiescents, with a few being visible for several solar rotations. They are most often found where there is little major activity such as the so-called “Polar Crown” high latitude areas, although they may sometimes be found between or even near active regions. The Hedgerow prominence is a fairly common QRF, and tends to look like an irregular row of bushes. Hedgerows tend to be highly detailed, with considerable branching and fine structure being visible at high resolution. QRFs appear in many other forms, including floating arches, fans, curtains, large curving blades, flames, and magnificent spiderweb-like arcs. Many have upper edges that tend to be sharper than their lower sides, which can be irregular or scalloped in form. Motion of material in QRFs is usually slow and small in scale, leaving the overall prominence shape unchanged, but minor changes can occasionally be seen in as little as 10 minutes. When viewed on the solar disk, QRFs tend to lose some of their fine detail, becoming faint irregular dark patches or large gently curving arcs of dark matter. Prominences are darker on the disk because they absorb light from below and then re-emit it in all directions. Some low-level filaments will often show a brighter base due to a “blanketing” effect they have which reduces the emission losses of the lower chromosphere. Large Quiescents are not always stable, and if they exceed a height of 50,000 km above the surface (0.07 solar radii), they will usually break loose from the sun within 48 hours in an eruption known as a “Disparition Brusque” (sudden vanishing). Disparition Brusque eruptions usually last less than one or two hours. They vary in form from a simple fading away of the quiescent, to a spectacular “lifting off”, where the prominence rises up and drifts away from the sun, slowly breaking up in the process. Mild surface brightening can also occasionally occur. Sometimes, a vanished quiescent will even reform a few hours or days later near its previous location. In a few large eruptions, a Dispartition Brusque can produce a Coronal Mass Ejection. Some prominences do rotate slowly, and a few even look and act like tornadoes, especially Disparition Brusque debris. Active Region Filaments (ARF’s) (best seen on the solar disk) tend to be smaller, darker, and narrower than their giant cousins, the QRFs. They are present in or near active regions and sometimes wind around or even through large sunspot groups. The Neutral Line Filament in an active region under- going the shearing of local magnetic fields is an example of an ARF. Arch Filament System “filaments” between forming sunspots are really fibrils, tending to be small and fairly short-lived unless flux emergence goes on for very long. They can be particularly dark when a spot is emerging, and often show Doppler shifts from material flowing in the fibrils. ACTIVE FILAMENTS/PROMINENCES are short-lived features associated with solar flares and other violent events. Limb Flares can appear on the solar limb as elevated bright blobs. Occasionally, a small ARF in which a flare begins will rise and expand into a majestic Eruptive Prominence, often in the form of a twisting loop, while intense emission appears on the surface. Flares sometimes produce a SURGE Prominence, visible near the limb as a well defined jet of bright gas, and which can occasionally be seen in emission on the solar disk.
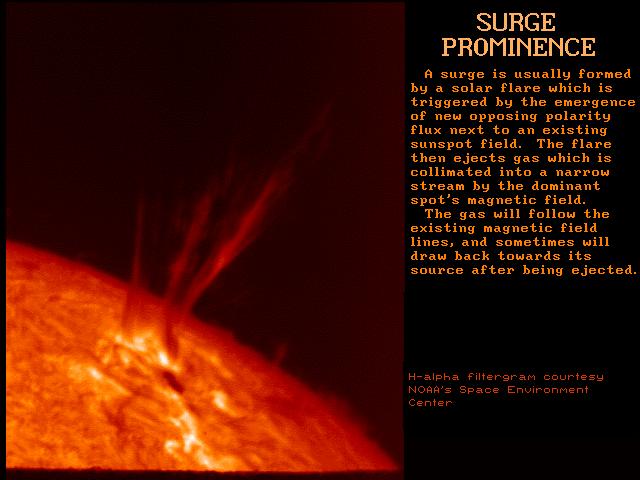
These jets sometimes take the form of fine closely-spaced spines which may be visible up to a solar radii from the limb. Surges can also be seen as bright-based narrow dark features on the disk of the sun (sometimes blue-shifted). Surges usually happen when a small satellite spot of opposite magnetic polarity comes up in or very close to the penumbra of a big regular sunspot. A small flare then occurs, resulting in an outward ejection of gas which is confined into a narrow stream by the dominant spot’s field. The surge ejecta’s velocity (50-200 km/sec) isn’t enough to leave the sun, and the gas will follow magnetic field lines as it falls or draws back, sometimes creating a “splash” of brightening near the point of origin. Much smaller non-flare surges can often be seen in active and occasionally in Ephemeral regions on the solar disk as tiny blue-shifted “smoke stack” or “puff” filaments, which resemble a smoke plume. Smoke stack surges usually last only a few minutes, and can occasionally occur in the middle of a fairly quiet area, especially in the early stages of an Emerging Flux Region. Another active prominence type, usually resulting from the most violent flares, is the SPRAY, a spectacular uncollimated expansion of disrupted ARF material, sending debris flying off in many directions at speeds of 200-300 km/sec or more (up to 2000 km/sec for some of the largest flares). Often, the filament involved becomes slightly elevated initially, then it explodes outward in the spray. Motion of spray material can often be seen in only a few minutes time, and occasionally, material can be seen leaving the sun entirely (Coronal Mass Ejection). Two other types of flare-associated active prominences are the Flare Loops, and Coronal Rain. FLARE LOOPS are large thin circular or elliptical vertical loops of gas which appear in the later stages or after a major flare. They tend to be brighter at the top and at their bases, and often show a nested structure of many very thin loops (Arcades), all with nearby endpoints. These loops are where flare ejecta or other gas has encountered the new magnetic field lines set up by the reconnection which occurs during the flare. Motion of material can often be seen down both sides of the loop towards the surface. The first loops form fairly low, while succeeding loops form higher and higher above the surface. Flare loops are sometimes not seen after some explosive flares or with small flares. They usually last only a matter of hours, and should not be confused with the quiescent “Floating Arches” prominences, which are thick or irregular in form, and are much longer lived. CORONAL RAIN is the diffuse faint patchy emission from gas descending back towards the surface as it encounters magnetic field lines. It can often be seen often as material “raining” down into post-flare loops or active regions.
COMMONLY VISIBLE CHROMOSPERIC DISK FEATURES (seen in filters with FWHM bandwidths under one angstrom) At the edge of the solar limb in H-alpha, the Chromosphere is seen in profile, appearing as an irregular fringe of red light less than 10 seconds of arc high, running all the way around the edge of the disk. At high power (especially in the wings of H-alpha), the individual Spicules making up this fringe are sometimes visible as tiny narrow jets of light, usually tilted slightly with respect to the vertical, and sometimes tending to blend together somewhat. Spicules are also visible on the disk as tiny narrow low contrast darker jets which tend to emerge from elements of the Chromospheric Network, an array of long sinuous chains of tiny slightly brighter patches which run over much of the solar disk. The Network is often difficult to see due to its low contrast and the ever-present overlying spicules, but is better shown in the wings of H-alpha, and is enhanced near active regions. Also hampering network visibility are the Fibrils, tiny low contrast short narrow filament- like darker features running between nearby points. Groups of longer fibrils which run directly between areas of opposite magnetic polarity are known as Field Transition Arches. Together, spicules and fibrils make up the Dark Mottles, which cover much of the solar disk, and which are often incorrectly referred to as the Network. Filaments are prominences seen against the solar disk and appear as dim patchy features or darker curving arcs. Quiescent filaments are generally larger than the somewhat narrower and more sinuous Active Region Filaments. Sunspots are visible in H-alpha, but their penumbras are lower in contrast than in white light. Frequently, fibrils will be seen near sunspots, tracing out the nearby magnetic field lines. Also visible at times in or near active regions is Plage; patchy areas of brightness marking nearly vertical emerging or rapidly realigning magnetic fields. Plage and white-light faculae are related, but are not the same thing, since they often don’t occupy exactly the same positions.
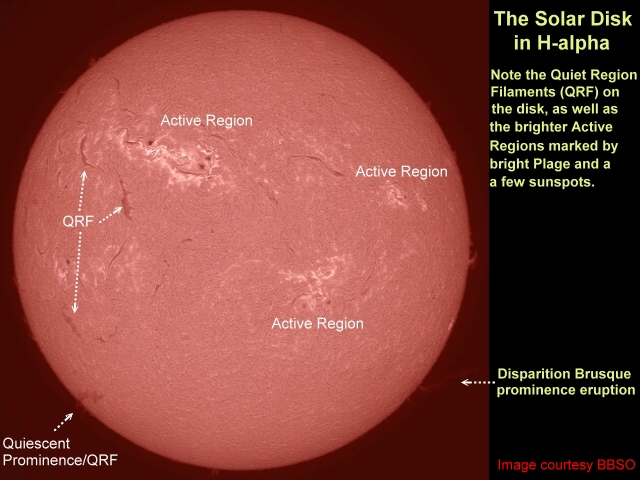
Active Region close-up:
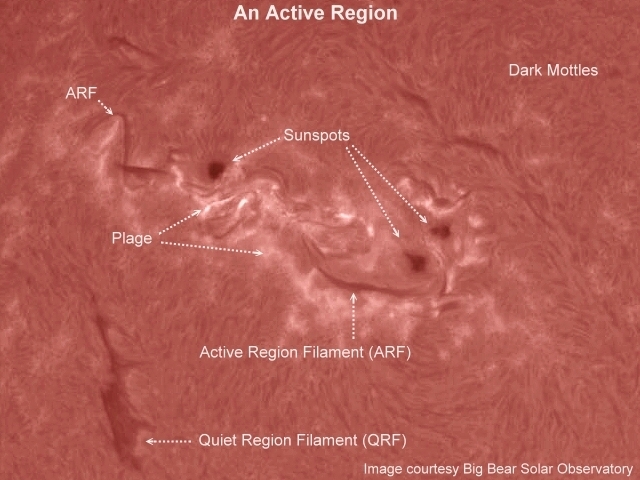
SOLAR ACTIVITY
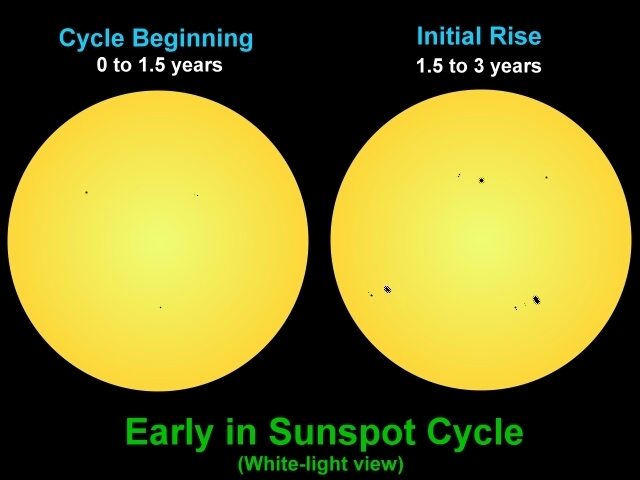
The number and magnetic polarity of sunspots varies according to an approximate 11 year numerical (22 year magnetic) cycle. About 18 months before the end of the old cycle, the first new cycle spots may begin to appear near 25 degrees north and south solar latitude, with the few remaining spots from the old cycle straddling both sides of the equator. After the old cycle spots die out, the new ones become more numerous and larger, forming distinct sunspot groups. These groups usually consist of a larger leader spot or spots, often followed as the sun rotates by several somewhat smaller trailing spots. Activity also spreads out in location, with a “jump” in sunspot formation latitude signaling the upturn in the new cycle. Each hemisphere eventually forms an irregular belt of spot activity that slowly drifts towards the equator as the cycle progresses.
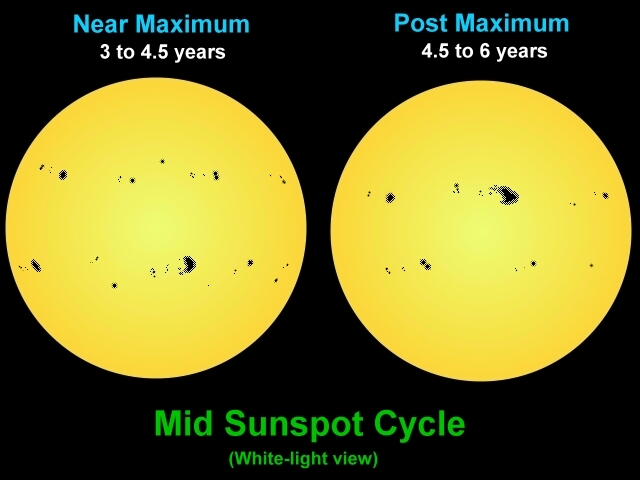
Near mid-cycle, sunspot number maximum usually occurs, with the main activity belts now being nearly 40 degrees wide, centered around 20 deg. N/S solar latitude (a few short-lived spots have been seen up to 70 deg. N/S). Very large complex groups of spots will be present near and after maximum, with many having complicated magnetic structure.
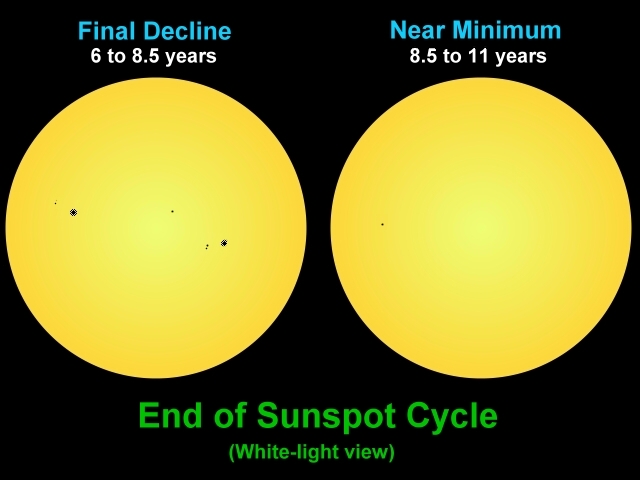
The number of spots then declines over the next few years, with most forming at lower solar latitudes and fewer large ones developing. As sunspot minimum again approaches, there are few if any spots visible, mostly near 7 deg. N/S. SUNSPOT GROUPS: Sunspots generally form in magnetically-linked bipolar groups, with each end being one pole of a localized magnetic field called a flux tube. The magnetic configuration of this flux tube (or “dipole”) is usually governed by the Hale-Nicholson Rules, which state that the preceding polarity spot is usually the dominant “leader” in most groups for the entire 11 year sunspot cycle. For example, in the northern solar hemisphere, the spots leading each group across the sun as the sun rotates (preceding or “p”) might start out one sunspot cycle having a “north” magnetic polarity. The followers (f) in the same group would then have a “south” polarity. Preceding spots in groups in the southern solar hemisphere would then have a south magnetic polarity and would be followed by the group’s north polarity spots. This polarity orientation of sunspot groups will generally be maintained until the next sunspot minimum, when polarities will reverse for both hemispheres. The magnetic axis of the sunspot group is usually slightly inclined to the solar east-west line (Joy’s Law), running from 3 degrees near the equator to 11 degrees at latitude 30 N/S, with the preceding polarity spot being slightly closer to the equator. If the axis is highly tilted initially, the group will tend to rotate until the axis is more parallel to the equator. P polarity spots in most bipolar groups tend to be a bit larger and better developed than the somewhat more numerous f polarity spots. P spots in developing groups also tend to move out westward to the head of the group. If a group starts out with the f polarity leading (“Inverted Polarity”), it will usually die out, or the p spot or the p polarity area behind the f spot will push westward through or past the field of the f spot, creating magnetic shear and possible flare activity until it regains its rightful place in the leading end of the group. Stable sunspots tend to be fairly symmetrical unless there is extensive magnetic shear nearby from emerging magnetic flux or the passing of an area of opposite magnetic polarity. Magnetic shearing can cause large portions of sunspot penumbras to distort or vanish. Large spots generally form from the merger of smaller ones. Large spot groups can be over 182,000 km long and usually result from the emergence of several flux tubes, since individual dipoles rarely exceed 50,000 km in length.
THE MT. WILSON MAGNETIC CLASSIFICATION OF SUNSPOT GROUPS Suffixes p or f are used when the preceding or following polarity spot, respectively, is dominant. ALPHA: A single dominant spot, often linked with a plage of opposite magnetic polarity. BETA: A pair of dominant spots of opposite polarity (Bipolar, ie: a leader and a follower). GAMMA: Complex groups with irregular distribution of polarities. BETA-GAMMA: Bipolar groups which have more than one clear north-south polarity inversion line. DELTA: Umbrae of opposite polarity together in a single penumbra. Just over half of the observed groups are Beta-p or Alpha-p, with the larger groups most often being Beta-p, Beta-Gamma, or Delta. Delta groups are generally very active and often are the site of major solar flares.
SUNSPOT GROUP DEVELOPMENT SEQUENCE: In white light, an early indication of a developing sunspot group is often the appearance of a few tiny pores, sometimes separated by a short distance, and possibly accompanied by faculae. In H-alpha, a bipolar group begins as a small bright oval area of plage with a few small surges. Several hours later, the pores and Arch Filament System (AFS) superimposed on very bright plage begin to appear, forming what is known as an Emerging Flux Region (EFR). The AFS often takes the form of several closely spaced fine filaments that arch between the emerging magnetic flux tube’s poles (often marked by pores), tracing out the field lines like those of a bar magnet. These AFS filaments show upward motion (blue shift) at the tops and downward flow at the ends (red shift). Small bright points of H-alpha emission lasting only a few minutes known as Ellerman Bombs can often be seen near the middle of the EFR. Rapid EFR growth then follows with some of the pores intensifying or merging to form the first sunspot umbras. Usually, the (p), or preceding polarity spot will form first and then move westward relative to the following (f) polarity spot (spot “Proper Motion”) to the eventual head of the group at about 1 km/sec. One to two days after formation, the first spots frequently form penumbras, their proper motion stops or slows, and the arch filaments connected to them usually disappear or erupt upward. The (p) spots may continue to move westward as long as new flux continues to emerge (this usually leaves some plage and a limited AFS near the center of the group where a few more pores or spots might eventually form). *Bright plage with arch filaments are good signs of continuing emerging magnetic flux.* (f) spots will either remain relatively fixed or will drift slightly eastward. In large active spot groups, the (f) spots may occasionally continue to develop until they rival the (p) spots in size. Sometimes, a (p) spot will develop accompanied by only bright plage and a few small filaments instead of trailing spots (Alpha group). Plage is rarely seen ahead of the (p) spots unless satellite opposite polarity is emerging. If small emerging flux regions emerge offset from the central AFS axis, a finger of plage forms extending to the new pole until its fields reconnect. If the EFR comes up deep inside a plage, the opposite polarity spot will be surrounded by Field Transition Arch fibrils connecting to the plage. Beta groups often form when only one or two adjacent flux tubes with the same orientation come up together. Extended groups can form from from the emergence of several dipoles end to end, sometimes yielding Beta-Gammas if polarities are mixed. Multiple flux tubes emerging fairly closely together in sequence or tilted to each other can form Gamma or Delta groups.
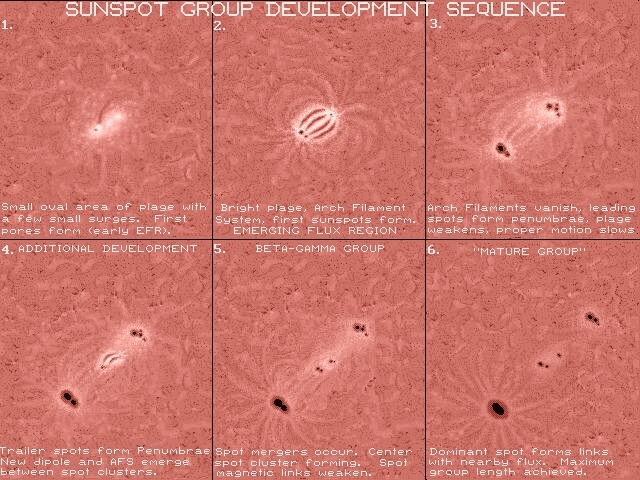
As magnetic flux emergence ceases, the individual spots of the dipole(s) frequently interact with local fields and other spots, behaving as if they had never been connected to each other. The main dominant spot will often form magnetic connections to distant fields, sometimes forming a “moat” around itself consisting of a thin patchy ring of brightening and a group of nearly radial H-alpha fibrils roughly twice the penumbral width. Once the active region becomes “mature” (reaches maximum longitudinal spread), it will tend to slowly decline and fade away unless more flux emerges to keep it alive. Mature spots rarely move very much, nor do they show much activity unless new flux emerges nearby. The spot motion that leads to magnetic shear and flares is almost always in an Emerging Flux Region. However, flux is somewhat more likely to emerge near where it has previously emerged, so even mature spots bear a little watching. Normal EFR development results in a fairly peaceful spot group which may have produced a few minor flares. After a few quiet weeks of existence, its spots usually shrink and decay into two diffuse unipolar areas, sometimes marked by weak H-alpha brightening and/or a filament. In declining groups, the smaller f polarity spots usually vanish before the dominant spots begin to decay. Most groups finally decay into a single p spot with no plage, which then slowly shrinks and dies out. DELTA GROUPS: Deltas are some of the largest and most active areas on the sun. The Delta is defined as two or more umbrae of opposite polarity which are inside a single penumbra or penumbral area. The opposite polarities are generally within two degrees of each other. Deltas usually form in one of three ways. 1. A single complex emerges at once with the dipoles intertwined and polarities reversed from the Hale-Nicholson rules (ie: (f) polarity leading (p)). This is sometimes known as an “Island Delta” group. 2. Large satellite polarity areas emerge close to existing spots so that the expansion of the emerging flux region pushes a p spot into an f spot or vice versa. 3. A growing bipolar spot group collides with another separate dipole so that opposite polarities are pushed together (this is the most frequent mode of delta group formation). It only forms from emerging umbrae, not plage. If the new dipole emerges into plage only, modest flares may occur without delta spot group formation. If it emerges under or collides with an umbra of opposite polarity, the delta spot forms and larger flares occur. If the dipole collides with an umbra of the same polarity, the two do not necessarily merge, but coexist peacefully. DELTA GROUP CHARACTERISTICS: Usually, Deltas show most of the following features: 1. Delta groups are almost always large, and 90% of those with inverted polarity have a high level of activity, especially in the occurrence of major flares. They often have a complex, irregular, or “broken” umbral look. 2. Delta groups form by joining opposite polarity spots from different dipoles, which are connected by sheared magnetic field lines, instead of direct (free space) lines of force. All the spots are inside the same penumbral area. 3. Delta spots rarely last more than one solar rotation and are somewhat shorter-lived than other spots of the same size (however, new delta spots may emerge in the same complex). 4. Delta spot polarity orientation is generally inverted as compared to the Hale-Nicholson Rules. 5. Delta spots do not usually separate, but die out locked together (rarely, umbrae are ejected from the group). 6. Active Delta regions are usually marked by bright H-Alpha emission especially when it occurs over umbrae marking continued flux emergence. Sometimes, a filament can be seen coming out of, or crossing the group.
SOLAR FLARES
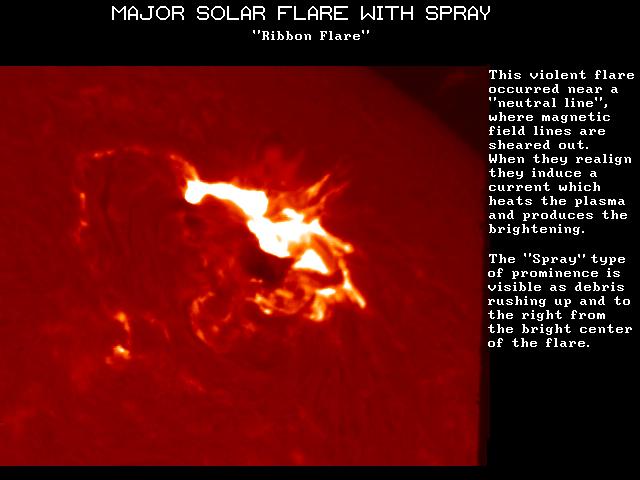
ORIGINS AND CLASSIFICATION: Flares are intense, abrupt releases of energy which occur in areas where the local magnetic field is rapidly realigning or changing because of magnetic field stress. This stress is usually induced by opposing magnetic flux emerging in or close to an existing active region. The new flux must either cancel the existing fields or push them aside. Since field lines imbedded in the solar plasma can rearrange only slowly in response to these changes, magnetic stress can build up to an extreme point, sometimes resulting in a flare. The older flux is pushed aside, creating strong gradients at the edges of the emerging field. As a new dipole emerges, a p spot usually forms and then moves forward at a rapid rate, squeezing any existing flux ahead of it. The new dipole’s field also expands in other directions, replacing the older field. As new flux pushes into existing flux of the opposite sign, there is some immediate realignment or “reconnection” of the fields (marked by H-alpha brightening), but as the material is pushed out of the way, the field lines are sheared or drawn out along the line perpendicular to the motion, and a Neutral Line is formed, defined as the sheared magnetic field boundary dividing regions of opposite magnetic polarity. Note: None of this happens if the moving spot pushes into flux of the same polarity. Also, the compressing and shearing of fields generally occurs only with moving sunspots and not with plage fields. A Neutral Line Filament sometimes forms along the neutral line, supported by horizontal sheared field lines. At high resolution, the filaments and fibrils in the area tend to be elongated and parallel to the neutral line, leading out to or from the main neutral line filament. Magnetic stress caused by this shearing builds to the breaking point, when a lower energy magnetic connection to material directly across the neutral line is possible. As this massive reconnection happens, magnetic energy is released in a flare, usually along or near the part of the neutral line where the stress is the highest. The rapid realignment of the magnetic fields induces intense electric currents which heat the plasma and produce extreme H-alpha brightening, which for flare brightness is defined as at least twice the normal chromospheric emission level. As the flare dies down, the sheared field line produced by the original motion and marked by the filament is replaced by loop prominences or field transition arches which arc more directly between two opposite polarity areas, tracing out the new field lines nearly perpendicular to the original neutral line boundary. At that point, the magnetic fields are connected in the lowest possible energy state and, unless further flux emerges, the flares should be over.
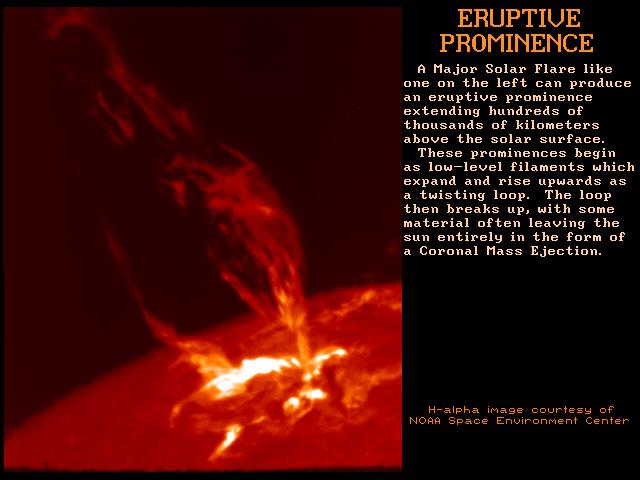
The frequency of solar flares is directly related to sunspot activity, with few occurring near sunspot minimum. Near sunspot maximum, small ones occur almost daily, and major flares can happen several times per week. Flare activity (and often intensity) tends to peak in the years near or just after sunspot maximum. Most solar flares occur in or close to growing or disturbed active regions, with the largest flares most often associated with Gamma and (especially) Delta spot groups. Solar Flares can often be grouped into two classes: Compact, and Major. Compact flares are usually smaller and somewhat more frequent than major flares. They often occur in a pre-existing loop or arch filament system, and little structural change in the area is observed. Compact flares can be seen in or near Emerging Flux Regions, and produce mainly small surges or none at all. Subflares are the smallest of the compact class, and are short-lived, being only slightly brighter that active plage. Major flares are considerably more violent and longer lived, frequently producing large surges or sprays of bright gas. They often emit intense X-rays and masses of energetic particles (Coronal Mass Ejections) that later can trigger geomagnetic disturbances on Earth. Major flares often cover large areas of the sun and can cause plage brightening or flare-like phenomena some distance away from the main event (electron brightening). A Moreton Wave produced by impulsive flares can sometimes be seen as a diffuse arc of brightening expanding outward across the solar disk. Moreton waves can occasionally disturb or disrupt some filaments which lie in their paths, sometimes making them vanish, only to reform later near their original location. Flares on the solar disk frequently show two areas of emission on either side of the magnetic inversion line, because energy released anywhere in a flux tube will rapidly heat the surface at its two footpoints where it meets the surface. When many lines of force are involved, two ribbons of emission (Two Ribbon Flare) appear. In great flares, the strands rapidly elongate on either side of the neutral line and separate at 5-20 km/sec while narrow flare loop prominences form to connect them, rising higher in the corona. If one ribbon is near a sunspot, it will be small and bright, because many flux lines converge there. The ribbons will not cross the spot since the other side involves magnetic field lines connected away from the flare. In the late stages, the strands evolve into two thin lines formed by the intersection of a thin shell of hot coronal material with the surface. Since reconnection means that two tubes of force interchange their end points, one expects four areas to brighten, and in larger flares these often can be picked out. A few flares will sometimes display only one or even three distinct ribbons instead of two or four, although the reason for this is unclear. Solar flares are ranked in importance by optical, X-ray, or radio flux. Soft X-ray intensity is measured in the 1-8 Angstrom range monitored by the GOES weather satellites. The classes are designated by the letters Bn (n x 10-7 w/m2), Cn (n x 10-6 w/m2), Mn (n x 10-5 w/m2), or Xn (n x 10-4 w/m2), where n is the integer for each power of ten. Thus a flare classed as M3 would produce a soft X-ray flux of .00003 watts per square meter. Optically, flares are ranked by the area in square degrees of heliocentric latitude they take up on the disk. A square degree at the center of the solar disk is 12,147 km on a side, or at the sun’s mean distance, each side of the square would be about 17 seconds of arc across. The optical class ranges from S (subflares) to 4 (largest).
AREA (sqr. deg) | OPTICAL CLASS | TYPICAL SOFT X-RAY CLASS |
2.0 or less | S (subflares) | C2 |
2.1-5.1 | 1 | M3 |
5.2-12.4 | 2 | X1 |
12.5-24.7 | 3 | X5 |
More than 24.7 | 4 | X9 |
*A suffix (f, n, b) is added if the brightness is faint, normal, or bright, based on a visual estimate. FLARE SEQUENCE: Some gradual H-alpha brightening may often precede many flares. *Frequently (especially in major flares), the neutral line filament (or another nearby Active Region Filament), rises tens of minutes before the flare; it may get exceptionally dark, blue-shifted, or broadened in H-alpha*. Then, the flare breaks out with brilliant H-alpha emission in what is known as the Flash Phase. Flare emission usually consists of three parts: small bright Kernels (often the first feature seen) where the H-alpha line is broad and the intensity is up to three times the photospheric continuum, an extensive area of narrower (approx. 1 Angstrom) emission directly involved in the main energy release, and bright post-flare loops connecting the two ribbons. As large flares erupt, the neutral line filament will often blow away, forming a spray, while in other cases, the filament either expands upward into a twisting loop-like eruptive prominence, or it breaks up with considerable twisting and turbulence at the start of the flare. In addition, material dispersed by a flare near the limb may be seen descending again as “Coronal Rain” after the flare dies down. A filament superimposed on plage or a sunspot will usually erupt in a flare because of the conflict between the nearly vertical plage/umbral magnetic field and the horizontal filament field. If the filament does not blow away, the area may flare again (homologous flares), since the magnetic shear stress is still present.
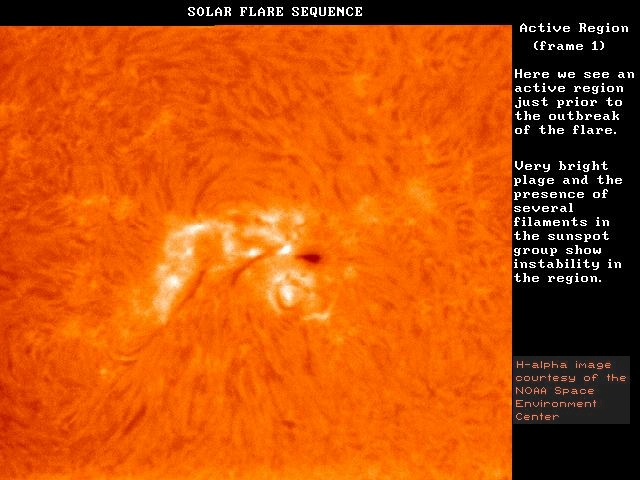
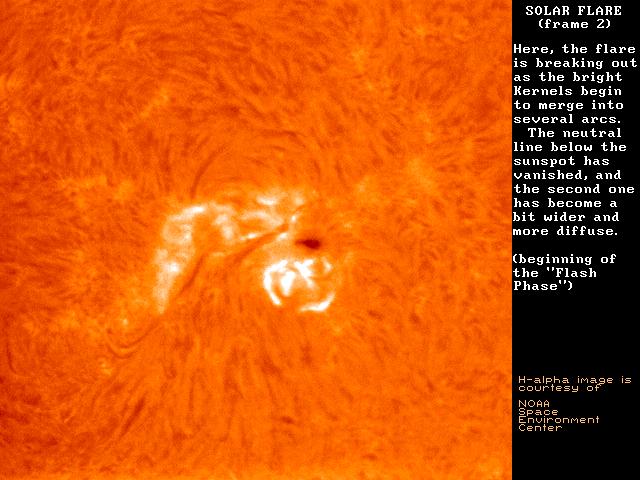
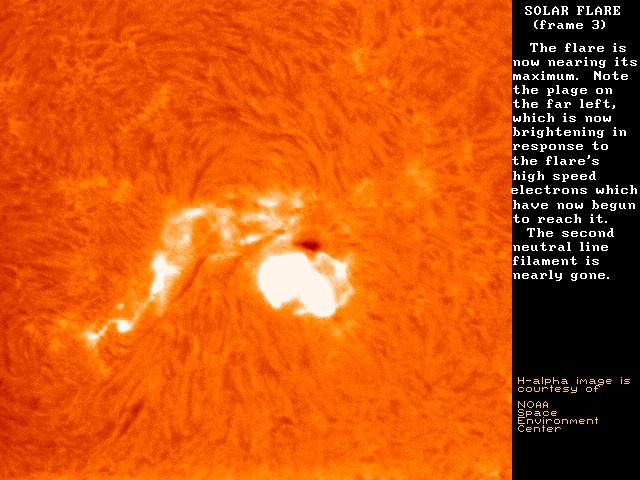
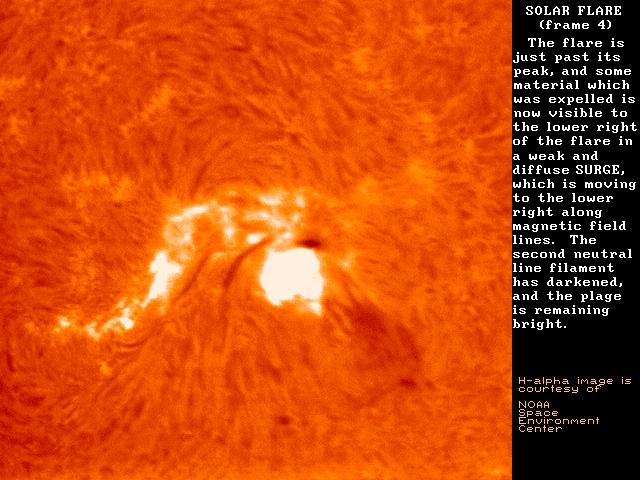
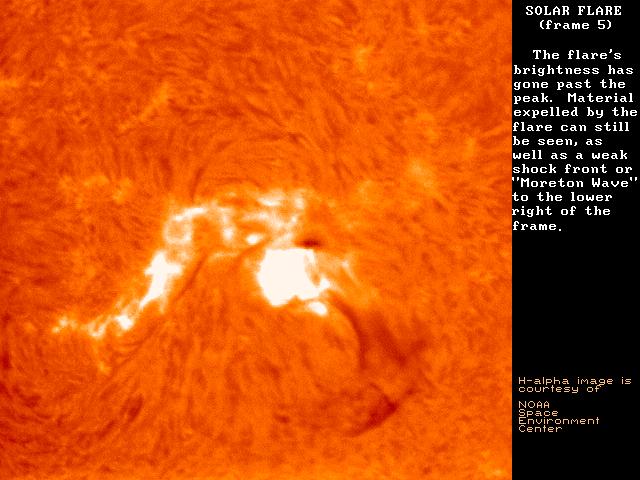
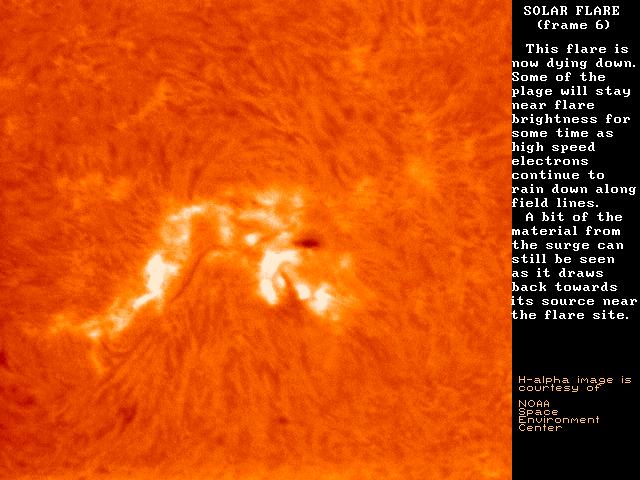
Frequently, a flare will occur towards the particular end of a netural line filament where magnetic flux conflict from moving sunspots is the greatest. Occasionally, the neutral line is not marked by any one distinct filament, or has a filament which is very narrow and difficult to see. This often happens when f polarity flux suddenly emerges just ahead of a well developed p spot. Then, the flares seem to come out of nowhere (sometimes producing a surge), however, they are still near a neutral line. Prior clear neutral line filaments may also not be easily seen when an EFR is rapidly replacing weaker existing fields, triggering compact or smaller flares. Most flares have a fairly rapid initial rise in brightness, approaching maximum intensity in only a few minutes. The brightness then stays high for a slightly longer period than the rise time before declining slowly. However, a few flares or flare-like phenomenon classed as Long Duration Events (LDEs) have a more gradual rise in brightness and are less impulsive, occasionally lasting up to 12 hours.
WHITE LIGHT FLARES: Intense flares which have bright emission over dark penumbrae or umbrae may occasionally be briefly visible (less than 10 min.) in white light as small bright patches. However, white light flares are rare. White light “light bridges” between umbrae are not white light flares, since they are a relatively long-lived purely photospheric phenomenon and only mark places where sunspot fibrils are weak or absent.
MAJOR FLARE PRECURSORS: Exact flare prediction is difficult at best. However, each of the following circumstances (alone or in combination), may indicate that a big flare may occur in the near future. 1. Delta groups, particularly those of origins 1 and 2. 2. Sunspot Umbrae obscured by H-alpha emission, or large umbrae without penumbrae. 3. Very bright H-alpha emission which marks flux emergence. 4. New flux erupting on the Leading side of the penumbra of a dominant p spot. 5. A filament crossing or closely encircling a delta spot group. 6. Strongly sheared magnetic configurations (inverted groups, strong elongation of umbrae (especially in pairs of opposite polarity), large-scale highly curved fibril alignment, ect.). SPOTLESS FLARES: Occasionally, flares will occur when a large filament imbedded in extensive plage left over from an old active region whose sunspots have decayed erupts. As the old fields decay, or a new EFR comes up underneath, the filament sometimes becomes unstable and erupts upward, producing brightening over a fairly wide area. They are not usually as violent as major flares, and little brightening occurs if the filament is very far from the plage (see Disparition Brusque eruptions in the prominences section).
SOLAR FEATURE PICTURE REFERENCES FROM ASTROPHYSICS OF THE SUN
ACTIVE REGIONS: p.163, 319, 327 CHROMOSPHERIC NETWORK: p. 156-7 DELTA GROUP: p.334 (flaring p. 359, and 345) DISK SURGE: p.299, 278 ELECTRON BRIGHTENINGS: p. 344 EMERGING FLUX REGIONS: p.172, 310-313*** (Note: the caption on page 310 actually refers to the pictures on page 313, and the caption on 312 refers to the pictures on p.310) ELLERMAN BOMBS: p.211, p.172-(fig. 7.11a in emerging flux regions), 314 ERUPTIVE PROMINENCE: p.269, 270, 295 FIELD TRANSITION ARCHES: p.163, 172-4, 310-313 HEDGEROW PROMINENCE: p.265-(limb), 268-(disk), 293-(disk over limb) FLARE LOOPS: p.282-3, 355 LIMB FLARE: p.272, 277, 354 LIMB SPRAY: p.280 LIMB SURGE: p.277 MORETON WAVE: p. 357 NEUTRAL LINES: p.174-5, 286, 338, 349-(flaring) QUIESCENT PROMINENCE: p. 267, 268 SOLAR FLARE: p.344-5, 349, 352-(near-limb), 359 SPICULES: p.161, 157, 159 SUNSPOT: p.2 (white light), p. 117-120 (granulation) TWO RIBBON FLARE: p.281 WHITE-LIGHT FLARE: p.360, 371(b)
FOR FURTHER SOLAR INFORMATION: Zirin’s book *Astrophysics of the Sun* is probably the best source for detailed information, and I highly recommended it, especially for its photographs. The text is fairly technical in places, containing much undergraduate and graduate-level Physics. The information relevant to the amateur tends to be a bit scattered throughout the book, so be prepared to do some searching. I make no absolute guarantees about the accuracy of all the information I have condensed and presented here. It is intended only as a general guide to benefit the amateur H-alpha observer, and may not be reproduced for profit.